1、Introduction
Lithium-sulfur (Li-S) batteries are recognized for their high theoretical energy density (2600 Wh kg-1) and cost-effectiveness of sulfur as an active material. However, their practical application remains hindered by critical challenges, particularly the notorious shuttle effect of lithium polysulfides (LiPSs) between electrodes, which leads to active sulfur loss and rapid capacity degradation. Developing highly efficient electrocatalysts to accelerate LiPSs conversion and suppress the shuttle effect is pivotal for advancing Li-S battery performance. Single atom catalysts (SACs) have demonstrated exceptional potential in optimizing sulfur redox kinetics and mitigating polysulfide shuttling. Nevertheless, existing studies predominantly focus on the active metal centers, whereas the co-catalytic interactions between SACs and their supports remain underexplored despite their crucial role in regulating sulfur redox processes.
2、Achievements
This study unveils the synergistic interplay between cobalt single atoms (Co-SACs) and graphitic carbon nitride (GCN) substrates, which significantly enhances sulfur reduction electrocatalysis. Density functional theory (DFT) calculations reveal robust interactions between Co-SACs and GCN, stabilizing the geometric and electronic configurations of the catalyst during reactions. The Co-GCN co-catalyst facilitates dual bonding with LiPSs via Co-S and Li-N bonds, effectively lowering the activation energy of sulfur reduction reactions (SRR) and suppressing polysulfide shuttling. Benefitting from such unique synergy, the battery demonstrates outstanding rate performance (718.9 mAh g-1 at 5.0 C) and yields a high areal capacity of up to 13.8 mAh cm-2 (1584.3 mAh g-1) under a high areal sulfur loading of 8.7 mg cm-2 but a low electrolyte/sulfur ratio of 5.0 μL mg-1. This study presents an effective approach to facilitate further investigation of SACs in enhancing Li-S battery performance by leveraging co-catalytic metal-support interactions.
3、Graphic and textual guidance
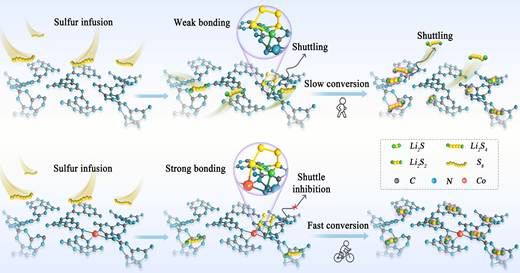
Scheme 1. Schematic of the conversion process of SRR on GCN and Co-GCN.
While GCN exhibits intrinsic catalytic activity by forming weak Li-N bonds with LiPSs, such interactions inadequately promote interfacial charge transfer. Introducing Co-SACs onto GCN creates abundant binding sites through N-Co frameworks, enabling simultaneous Li-N and Co-S bonding. This configuration strengthens LiPSs adsorption, reduces SRR energy barriers, and effectively mitigates the shuttle effect.
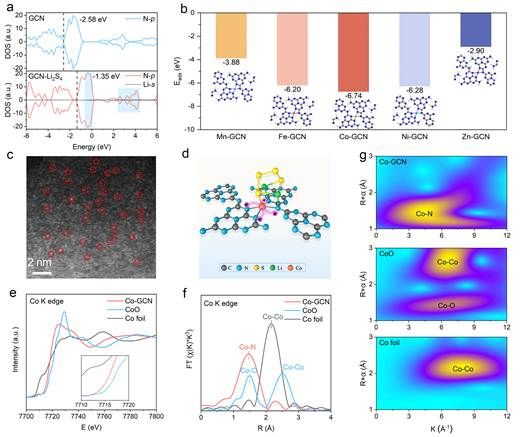
Figure 1. Theoretical screening, morphology, and structural characterization of Co-GCN
When Li2S4 adsorbs onto GCN, the p-band center of N atoms shifts toward the Fermi level, indicating enhanced electronic interaction between N atoms and Li2S4. The coupling between N p-orbitals and Li s-orbitals at the Fermi level confirms GCN's intrinsic catalytic activity in promoting Li2S4 adsorption and conversion. The binding energy between metal atoms and the support is a key factor determining the catalytic activity of SACs. Comparison of binding energies between different transition metals and GCN shows that Co-GCN exhibits the most stable structure. HAADF-STEM demonstrates the dispersed state of Co atoms. XANES spectra reveal that Co in Co-GCN displays a higher oxidation state. The characteristic peak at 1.4 Å in EXAFS corresponds to Co-N coordination in the first coordination shell, with a coordination number of 4. Wavelet transform analysis confirms the isolated Co-N4 configuration in Co-GCN without any metal-metal bonds, demonstrating the successful synthesis of Co-SACs on the GCN support.
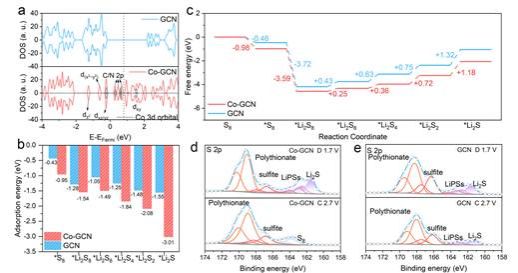
Figure 2. DFT and XPS investigations on LiPSs conversion promoted by Co-GCN
Density of states (DOS) analysis of Co-GCN reveals significant contributions from Co near the Fermi level, which enhances the electron-accepting/donating capability of Co-N4 sites. This electronic configuration facilitates faster electron transfer to lithium polysulfides (LiPSs), thereby promoting the sulfur reduction reaction (SRR). The strong binding energies of Co-GCN with S8 and various Li2Sx (x = 8, 6, 4, 2, 1) species demonstrate its effective suppression of the polysulfide shuttle effect. Furthermore, the smaller ΔG values in Gibbs free energy calculations indicate superior thermodynamic stability of SRR on Co-GCN. This enhanced reduction capability originates from strong d-p orbital coupling between Co and S atoms, which significantly lowers the energy barrier for LiPSs conversion. XPS studies confirm that the co-catalytic effect of Co-GCN promotes Li2S decomposition during charging, effectively preventing the formation of inactive "dead sulfur" on the catalyst surface that would otherwise lead to deactivation.
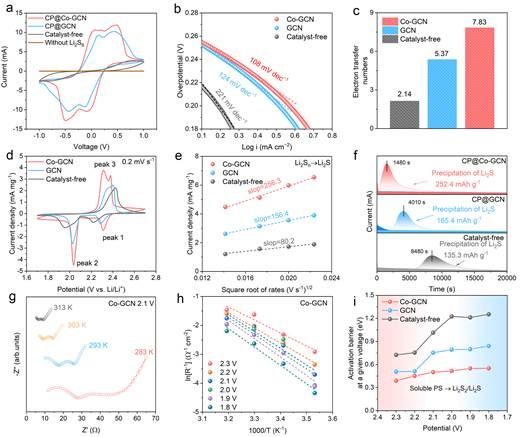
Figure 3. Catalytic reaction kinetics of LiPSs on Co-GCN
Electrochemical tests confirm the catalytic activity of GCN in Li-S batteries. In Li2S6 symmetric cells, GCN shows clear redox peaks and improves the electron transfer number in SRR, demonstrating its intrinsic catalytic activity. After loading Co single atoms onto GCN, multiple kinetic parameters show significant enhancement: the Tafel slope decreases, the electron transfer number increases, CV curves show reduced polarization and higher peak currents, lithium-ion diffusion coefficients improve, Li2S deposition capacity rises, and the activation energy for the rate-determining step of SRR decreases. These results prove the markedly improved catalytic activity of Co-GCN.
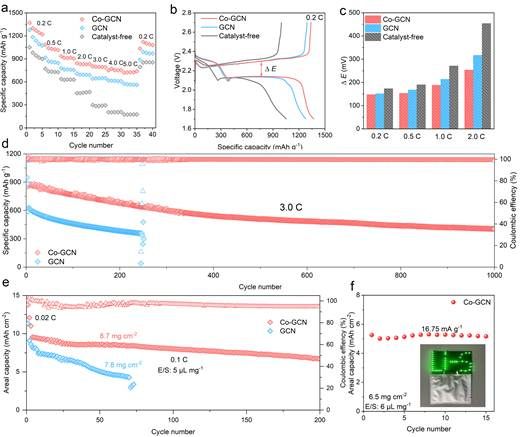
Figure 4. Electrochemical performance
Benefiting from the co-catalytic effect of Co-GCN, the Li-S batteries demonstrate outstanding rate capability (718.9 mAh g-1 at 5.0 C) with minimal polarization voltage differences across various current rates. Moreover, the batteries maintain stable cycling over 1000 cycles at 3.0 C, exhibiting an ultralow capacity decay rate of merely 0.05% per cycle. Under challenging conditions of high sulfur loading (8.7 mg cm-2) and lean electrolyte (5 μL mg-1), the batteries achieve a remarkable areal capacity of 13.8 mAh cm-2 (1584.3 mAh g-1) while retaining 70.5% capacity after 200 cycles at 0.1 C. Notably, a pouch cell employing Co-GCN modified separator maintains 98.3% capacity retention after 15 cycles at 0.01 C, demonstrating its practical application potential.
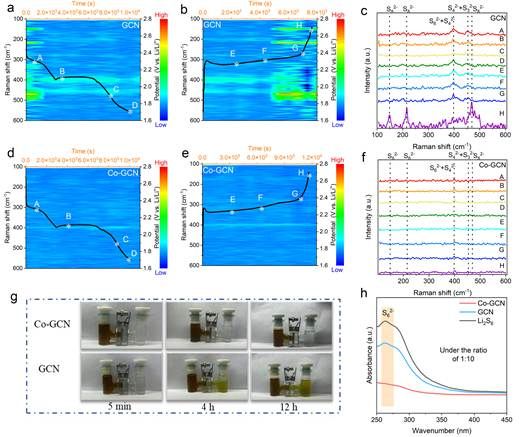
Figure 5. In situ Raman spectra and H-type electrolytic cells
To confirm the shuttle inhibition by using the Co-GCN catalyst, the in situ Raman spectroscopy was conducted to monitor the highly soluble LiPSs during battery operation. By monitoring Raman spectra during different charge/discharge states, it was found that the introduction of Co-GCN co-catalyst significantly reduced LiPSs shuttling in the battery and improved sulfur utilization. Further investigation using an H-type electrolytic cells device revealed the inhibitory effect of Co-GCN-modified separators on LiPSs shuttling. When using GCN separators, the electrolyte in the right chamber turned from colorless to yellow after 4 hours, whereas with Co-GCN separators, the electrolyte remained nearly colorless, demonstrating that Co-GCN can effectively suppress LiPSs shuttling.
Finally, the authors present conclusions and future perspectives. This study develops a strategy for loading single-atom catalysts onto intrinsically active GCN substrates, investigating the co-catalytic mechanism of single atom-support interactions in sulfur conversion. The results demonstrate that co-catalysis can optimize the SRR process and effectively suppress polysulfide shuttling between electrodes. Specifically, the interaction between Co-SACs and the GCN support facilitates the formation of both Li-N and Co-S bonds, which enhances binding with LiPSs, reduces the energy barrier for SRR conversion, minimizes LiPSs accumulation in the electrolyte, and improves sulfur utilization efficiency. Consequently, this approach significantly enhances the rate capability and cycling stability of Li-S batteries. This study presents an effective approach to facilitate further investigation of SACs in enhancing Li-S battery performance by leveraging co-catalytic metal-support interactions.